The announcement by the LIGO and VIRGO collaborations of direct detection of gravitational waves confirms one of Einstein’s major predictions, nearly one hundred years since it was made. Einstein submitted his paper with the correct equations of general relativity on November 25th 1915, and by June of 1916 he had already submitted a paper exploring the possible existence of gravitational waves. Something that is impossible in Newtonian theory.
We have a completely new window opened to look at the universe. The 21st century will be the century of gravitational wave astronomy. A new golden age is beginning. Not only because of the many phenomena that we expect to study, but also for those that almost certainly we had no idea of their existence.
In general relativity massive objects bend spacetime, and its curvature determines the motion of objects in that geometry. When massive objects move, the curvature of the geometry must change accordingly, and it takes spacetime some time to react. There are thus perturbations in spacetime that can be described by waves. Very similar to earthquakes, which generate waves on the earth crust, gravitational waves are like seismic waves in the fabric of spacetime. Since gravity cannot be screened, these waves travel throughout the universe.
One of the major difficulties in the detection of such waves is that they are very weak. The fractional change in the geometry can be as small as 10−21, and it is a feat of ingenuity to be able to detect them. This is like measuring the distance Earth-Moon with a precision of a few hundred atomic nuclei.
The merging of black holes is the cleanest way to produce gravitational waves. Black holes have occupied astrophysicists for decades, and there are heated debates about the nature of their interior, or the nature of the horizon that shrouds the spacetime singularity that may lurk in their interiors. These issues may finally be accessible experimentally. Many properties of black holes will be tested, but also the validity of Einstein’s theory in regimes where the gravitational interaction is very strong, and defies frequently our computational power. It is very exhilarating to envisage the possibility of opening the experimental age in black hole physics. We will indeed live very exciting times.
The first indirect detection of gravitational waves is due to Russell Hulse and Joseph Taylor (Nobel Prize 1993) due to the study of the binary pulsar PRS B1913+16 discovered in 1974. One of the elements of the pair is a pulsar. By carefully analysing the timing of the signal they announced in 1978 the direct measurement of the decay of the orbit due to the emission of gravitational waves according to Einstein’s famous quadrupole formula. Better yet, in 2003 a double pulsar was discovered (PSR J0737-3039) with an orbital period of 2.4 hours. In this case both elements of the pair are pulsars. Once again, the gravitational decay of the orbit was measured with exquisite precision, and in agreement with general relativity.
There are many sources of gravitational waves which can be seen in the picture below:
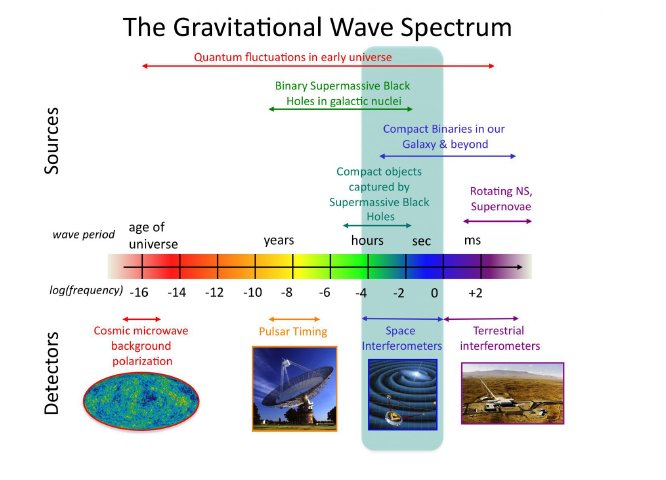
- Extremely low frequency. The wavelengths have cosmological scale, and they can be probed with satellites measuring the B-mode polarization of the cosmic microwave background radiation.
- Gravitational radiation with a wave periods of a few years could in principle be measured by observing an array of millisecond pulsars. Several projects are currently monitoring pulsars in search for gravitational wave signals.
- Low to moderate frequencies. There are many sources in this range (see graphic), whose detection would require space interferometers. So far the satellite LISA pathfinder has been launched a few months ago, and it will test some of the technology necessary to build such instruments. Launching of a full LISA (Laser Interferometer Space Antenna) is not planned so far till the mid 2030s.
It is in the high frequency range that ground based interferometers like LIGO and VIRGO are useful. The possible sources include mergers of compact objects: neutron stars, black holes. Black hole mergers provide the cleanest signals. It is believed that such mergers happen with a frequency of the order of one per galaxy per thousand years. This is one of the reasons why having the sensitivity to reach to the VIRGO cluster of galaxies is crucial. There are nearly 2000 galaxies there, so the number of galaxies that can be monitored by current interferome- ters is in the many thousands, hence the probability of having several detections per year is high.
Further comments:
-
The best known black hole solution was obtained by Karl Schwarzschild (and it carries its name), who sent it to Einstein in a letter dated at the end of December of 1915, hardly one month after he presented the final form of the equations of the gravitational field. It is the more remarkable since Schwarzschild was in the Russian front. One should also admire the efficiency of the Prussian mail office. . .
-
The first analytic study of black hole formation as a consequence of star collapse was done in 1939 by Oppenheimer (yes, that one) and Snyder.
-
It took till 1963 to obtain the expression of the spacetime representing a rotating black hole, and this was due to Roy Kerr. Soon after the Kerr-Newman solution of general relativity was obtained representing a rotating and electrically charged black hole.
-
The existence of gravitational waves as a prediction of general relativity was a very controversial subject for decades, and it was not settled that they exist and carry energy until the late 1950s, thanks to the work of Pirani, Bondi and Feynman.
-
Their is a large body of theoretical work dedicated to the study of black holes, singularities in general relativity and the interplay with quantum mechanics. Some of the major players are: S. Chandrasekhar (Nobel 1983), J.M. Bardeen, S. Hawking, R. Penrose, B. Carter, J. Wheeler, K. Thorne, C. Misner, V. Belinsky, I. Khalatnikov, E. Lifshitz, L. Landau, J. Hartle, G. Gibbons, J. Bekenstein, W. Unruh...